Jump to a key chapter
Material Strength and Mechanical Properties of Materials
Understanding the material strength and mechanical properties of materials is crucial for engineering and design applications. These properties determine how materials react to external forces and their ability to withstand them without deforming or breaking.
Definition of Stress and Strain
The concepts of stress and strain are fundamental in evaluating material strength. Stress refers to the internal force per unit area within a material. It applies when an external force is applied. This is mathematically represented as \[\text{Stress} = \frac{F}{A}\]where \(F\) is the force applied and \(A\) is the area. The unit for stress is usually Pascal (Pa) or Newton per square meter (N/m²).Strain, on the other hand, is the measure of deformation representing the displacement between particles in the material body. It is defined as the change in length divided by the original length, represented by:\[\text{Strain} = \frac{L - L_0}{L_0}\]where \(L\) is the final length and \(L_0\) is the original length. Strain is a dimensionless quantity as it is a ratio.
Stress: The internal force per unit area within a material subjected to an external force. It is expressed in Pascals (Pa).Strain: A measure of deformation, calculated as the ratio of the change in length to the original length of a material.
For example, if you apply a force of 1000 N evenly over an area of 0.5 m² on a metal rod, the stress can be calculated as;\[\text{Stress} = \frac{1000}{0.5} = 2000\text{ Pa}\]If the rod originally measured 2 m and elongated to 2.1 m, the strain can be calculated as:\[\text{Strain} = \frac{2.1 - 2}{2} = 0.05\]
Remember that while stress has units, strain is a dimensionless number, and both are crucial to understanding material deformation.
What is Yield Strength of Material
Yield strength is a critical property for materials as it defines the stress at which a material begins to deform plastically. Before reaching the yield point, a material will deform elastically and return to its original shape when the stress is removed — beyond this, however, permanent deformation occurs. It provides engineers with the capacity to design structures and components within safe limits under applied loads.The yield strength can be represented by a stress-strain curve, where the point at which the curve begins to deviate from linearity indicates the yield strength.Mathematically, it is calculated when the stress applied equals the proportional limit stress.
Consider a steel beam used in construction that has a yield strength of 250 MPa. This value informs engineers that if the stress applied to the beam exceeds 250 MPa, it will not return to its original shape, potentially causing structural failure.
Understanding yield strength involves diving into the details of a material's behavior under stress. The nuances of different materials' stress-strain curves can be fascinating. For instance, some materials, like aluminum, have an obvious yield point, making it easier to determine. Meanwhile, materials such as titanium have more gradual transitions, requiring careful analysis to obtain accurate results.Moreover, understanding yield strength is crucial in industries like automotive and aerospace, where material failure can have catastrophic consequences. Engineers often consider safety factors in their designs, accounting for unexpected stresses or loads that could exceed the material's yield strength.
Material Strength vs Toughness
In engineering, understanding the distinction between material strength and toughness is essential. Material strength assesses the ability of a material to withstand forces without breaking, while toughness measures the ability to absorb energy and deform without fracturing.
Compressive Strength of Materials Explained
Compressive strength is a key characteristic of materials, especially those used in construction and structural applications. It refers to the ability of a material to resist deformation and failure under compression. Compressive strength is quantified by applying a compressive force to a material specimen and measuring how much it can withstand before it fails.Mathematically, compressive strength is expressed as: \[\text{Compressive Strength} = \frac{F_c}{A}\] where \(F_c\) is the compressive force applied and \(A\) is the cross-sectional area of the specimen. The result is typically measured in Pascals (Pa) or Megapascals (MPa).
Compressive Strength: The capacity of a material to withstand loads tending to reduce size. Measured as the maximum stress a material can withstand under crushing pressure.
Consider a concrete cube with a cross-sectional area of 0.25 m² subjected to a load of 200,000 N. The compressive strength of the cube would be calculated as follows: \[\text{Compressive Strength} = \frac{200,000}{0.25} = 800,000\text{ Pa} \] This value helps in determining the suitability of concrete for various applications.
Remember that compressive strength is crucial for materials used in construction, like concrete and stone, where the structural integrity is a priority.
Compressive strength testing is one of the most common and valuable methods of assessing the durability and reliability of building materials. Different materials exhibit different behaviors under compression. For example, metals tend to plastically deform, absorbing significant energy, whereas brittle materials, such as ceramics, may fracture suddenly when their compressive strength is exceeded. Engineers use standardized testing methods, such as the ASTM standards, to ensure that results are consistent and reliable. This kind of testing not only provides insights into a material's suitability for various applications but also helps in predicting how it might behave in unexpected scenarios, such as seismic activity.
Application of Tensile Strength in Engineering
Tensile strength is a crucial mechanical property that engineers consider when selecting materials for various applications. It represents a material's ability to resist forces that attempt to pull it apart. This property is vital in industries ranging from construction to aerospace, where materials must withstand significant tension without failing.
Understanding Tensile Strength
Tensile strength is measured by applying a force to a material until it breaks. The maximum stress it withstands during this process is its tensile strength. Engineers often use tensile testing machines to determine this property, providing valuable insights into a material's behavior under stress.
Tensile Strength: The maximum stress a material can withstand while being stretched or pulled before failing or breaking.
For example, consider a steel rod with a diameter of 10 mm subjected to a tensile force of 50,000 N. The tensile strength of the rod can be calculated using the formula:\[\text{Tensile Strength} = \frac{F}{A}\]where \(F\) is the force and \(A\) is the cross-sectional area calculated as \(\pi\times\left(\frac{d}{2}\right)^2\).Substituting the values:\[A = \pi\times\left(\frac{10}{2}\right)^2 = 78.54\text{ mm}^2\]\[\text{Tensile Strength} = \frac{50,000}{78.54} = 636.62\text{ MPa}\]
Materials with high tensile strength are ideal for applications where pulling forces are predominant, such as cables and bridge components.
Applications in Construction
In construction, tensile strength plays a pivotal role in determining the durability and safety of structures. Materials like steel and reinforced concrete, renowned for their high tensile strength, are often chosen for building bridges, skyscrapers, and other infrastructure.
Steel cables used in suspension bridges are meticulously engineered to possess high tensile strength. These cables must support massive loads while resisting elongation. The tensile strength ensures that the structure can withstand environmental forces such as wind and weight loads.
Applications in Automotive and Aerospace Industries
The automotive and aerospace sectors heavily rely on materials with excellent tensile strength to ensure safety and performance. Components like car frames, aircraft fuselages, and wings endure significant tension during operation, necessitating the use of materials that won't succumb to stress under extreme conditions.For instance, aerospace engineers utilize advanced composites and high-strength alloys to withstand the dynamic forces experienced during flight, optimizing the weight-strength ratio for enhanced fuel efficiency without compromising structural integrity.
Delving deeper into tensile strength, it's fascinating to consider how innovative materials are developed to achieve optimal performance in challenging environments. High-performance fibers used in making bulletproof vests, for example, exhibit exceptionally high tensile strength, offering protection and flexibility. Similarly, carbon nanotubes, though still primarily in research stages, hold immense potential for future engineering solutions due to their extraordinary tensile strength, being several times stronger than steel on a per-weight basis.
Real-World Applications of Material Strength in Engineering
Material strength is a core concept in engineering, influencing the choice of materials across a wide range of industries. Engineers heavily rely on understanding the mechanical properties of materials to design safe, effective, and economical structures. Applications of material strength permeate various fields, each leveraging its unique attributes to solve complex challenges.
Construction Industry Utilization
In the construction industry, selecting materials with appropriate compressive and tensile strength is crucial for the safety and longevity of structures. High-rise buildings, bridges, and tunnels are constructed using materials like steel and concrete to ensure stability and resistance to external forces.For instance, the use of reinforced concrete, which combines the tensile strength of steel with the compressive strength of concrete, is common in building is essential for withstanding a range of loads and environmental conditions.
Material | Type of Strength | Example Applications |
Steel | Tensile | Rebar, bridge cables |
Concrete | Compressive | Foundations, columns |
Composite Materials | Mixed | Structural frames, roofing |
For example, in the context of bridges, engineers apply the following principle:The ability of a bridge to withstand loads can be calculated considering both tensile and compressive forces. If a bridge designed to carry a load of 5000 N faces a tensile force, engineers must ensure the cables have a tensile strength greater than:\[\text{Tensile Strength Required} > \frac{5000}{A}\]where \(A\) is the cross-sectional area of the cable.
In construction, always verify material specifications with regulatory standards to ensure they meet the required strength and safety criteria.
Aerospace Engineering Applications
Material strength is equally vital in aerospace engineering, where optimizing the weight-to-strength ratio is crucial for aircraft and spacecraft. Engineers use advanced materials such as titanium alloys and carbon fiber composites to lower weight without sacrificing strength and safety.The high tensile strength of these materials ensures structural integrity and performance even under significant stress during launch and flight.
Tensions in aerospace materials often reach remarkable levels due to factors like gravitational forces and aerodynamic challenges. The development of materials with tailored properties, such as superalloys, allows for advanced performance. These materials are designed to perform well under extreme temperature and stress conditions. Understanding fatigue behavior and employing finite element analysis (FEA) to simulate real-world conditions are critical for engineers when developing aerospace applications.FEA helps engineers predict how materials will behave, optimizing designs to prevent failure and enhance performance. This technique involves breaking down complex structures into smaller parts, solving for stresses and strains in each component.
Automotive Industry Implications
In the automotive industry, the emphasis on material strength contributes to vehicle safety and efficiency. Using materials with precise mechanical properties can reduce the overall weight of vehicles, enhancing fuel efficiency without compromising safety standards.High-strength steel, aluminum alloys, and composites are commonly used for body frames, bumpers, and engine components to achieve these goals.
In a car's design, frontal impacts are a significant consideration. Engineers calculate the crumple zone's effectiveness by examining:\[\text{Impact force} = \text{mass} \times \text{deceleration}\]If a car with a mass of 1500 kg experiences a deceleration of 15 m/s², the impact force should not exceed the tensile limits of the material designed for energy absorption in the crumple zones. Advanced materials effectively disperse forces, minimizing passenger risk.
material strength - Key takeaways
- Definition of Stress and Strain: Stress is the internal force per unit area within a material, measured in Pascals (Pa). Strain is the ratio of change in length to the original length, a dimensionless quantity representing deformation.
- What is Yield Strength of Material: It is the stress at which a material begins to deform plastically. Before this point, materials deform elastically and return to their shape when stress is removed.
- Material Strength vs Toughness: Material strength is the ability to withstand forces without breaking, while toughness is about absorbing energy and deforming without fracturing.
- Compressive Strength of Materials Explained: Compressive strength is the capacity to withstand axial loads that reduce size, crucial for construction materials like concrete. Measured in Pascals (Pa).
- Application of Tensile Strength in Engineering: Tensile strength is vital for materials needing to resist tension, important in industries like construction, aviation, and automotive.
- Mechanical Properties of Materials: Understanding material properties like tensile, compressive, and yield strength is crucial in engineering to predict how materials respond to forces and design safe structures.
Learn faster with the 12 flashcards about material strength
Sign up for free to gain access to all our flashcards.
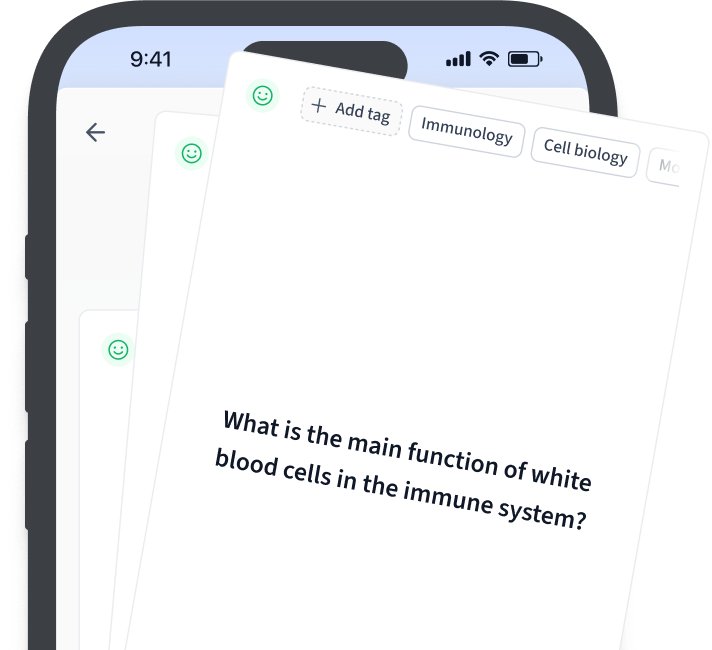
Frequently Asked Questions about material strength
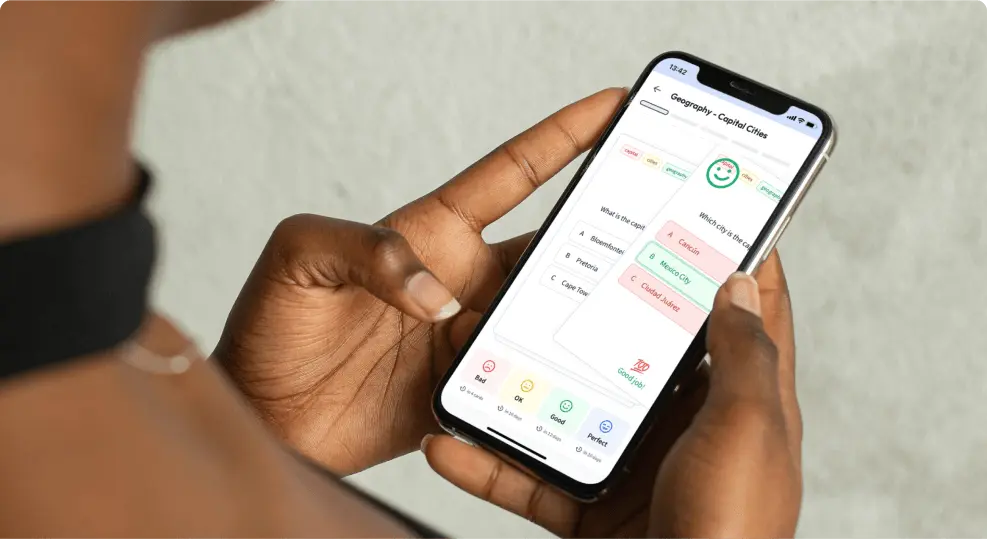
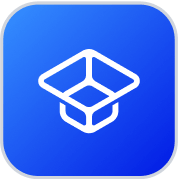
About StudySmarter
StudySmarter is a globally recognized educational technology company, offering a holistic learning platform designed for students of all ages and educational levels. Our platform provides learning support for a wide range of subjects, including STEM, Social Sciences, and Languages and also helps students to successfully master various tests and exams worldwide, such as GCSE, A Level, SAT, ACT, Abitur, and more. We offer an extensive library of learning materials, including interactive flashcards, comprehensive textbook solutions, and detailed explanations. The cutting-edge technology and tools we provide help students create their own learning materials. StudySmarter’s content is not only expert-verified but also regularly updated to ensure accuracy and relevance.
Learn more