Jump to a key chapter
Catalyst Life Cycle Definition
Understanding the catalyst life cycle is essential for students exploring chemical and process engineering. Catalysts are substances that speed up chemical reactions without undergoing permanent change themselves. However, these catalysts have their own life cycles which involve various stages from activation to eventual deactivation.By comprehending this concept, you enable a more efficient design of chemical processes and enhance the sustainability of catalytic reactions.
Stages of Catalyst Life Cycle
The catalyst life cycle can be divided into several key stages:
- Activation: In this stage, catalysts are prepared and activated to become operable. It involves treating the catalyst so that it achieves its maximum potential performance.
- Utilization: Once activated, the catalyst is used in chemical reactions. This is where the catalyst exhibits its properties, facilitating reactions by providing an alternative reaction pathway with a lower activation energy.
- Deactivation: Over time, catalysts lose their effectiveness and become deactivated. This can be due to factors such as sintering, poisoning, or fouling.
- Regeneration: Sometimes, deactivated catalysts can be regenerated to restore their activity level. Regeneration processes might involve chemical treatments or thermal processes.
Catalyst life cycle: A concept describing the different phases a catalyst goes through in its operational lifetime, including activation, utilization, deactivation, and regeneration.
Imagine you are working with a platinum catalyst in an industrial application. Initially, the catalyst is inactive. Upon heating, it becomes activated and facilitates the desired chemical reaction, such as the conversion of carbon monoxide into carbon dioxide. After extended use, you notice the reaction rate drops significantly due to catalyst deactivation, prompting a regeneration procedure to restore its activity.
In the deactivation stage, monitoring catalyst performance closely is essential, as it directly impacts the efficacy of the chemical reactions involved.
Deactivation can be investigated more mathematically. The rate of deactivation might be modeled using kinetic equations. For example, if a catalyst deactivates following first-order kinetics, the rate of deactivation can be expressed as:\[ - \frac{dC}{dt} = k_d C \]where C is the concentration of active sites, t is time, and k_d is the deactivation rate constant. This relationship helps in predicting how quickly a catalyst will lose its activity and informing regeneration strategies.On the flip side, understanding regeneration cycles is crucial for maximizing the lifespan of a catalyst. Regeneration is not always 100% efficient, often modeled by efficiency factors that determine how much active site concentration is restored after each cycle. This requires a comprehensive understanding of chemical engineering principles and access to precise control mechanisms for application in industrial settings.
Catalyst Life Cycle Explained
Examine the intricacies of the catalyst life cycle, an essential topic in chemical and process engineering. Catalysts, although not consumed in reactions, undergo distinct phases from activation to deactivation.
Key Phases in Catalyst Life Cycle
The life cycle of a catalyst is divided into the following stages:
- Activation: Catalysts are prepared to be reactive, often requiring specific treatments like heating.
- Utilization: The active catalyst participates in reactions, lowering the activation energy and increasing reaction rate.
- Deactivation: Gradually, catalysts lose activity due to poisoning or structural changes.
- Regeneration: Certain catalysts can be restored through chemical or thermal means.
Consider a catalytic converter in a vehicle: initially activated post-manufacturing, used over time to reduce emissions by converting pollutants into less harmful substances. Eventually, it becomes less effective due to deactivation from accumulated deposits. Periodic regeneration or replacement is then required to restore functionality.
Regular monitoring can help detect early signs of catalyst deactivation, optimizing maintenance schedules and improving longevity.
Delving deeper, the mathematical modeling of catalyst deactivation provides valuable insights into the process. For instance, assuming first-order deactivation kinetics, the rate of change of catalyst concentration can be expressed as:\[ \frac{dC}{dt} = -k_d C \]where C represents catalyst concentration, t is time, and k_d is the deactivation constant.Additionally, if you consider regeneration efficiency, equations become more complex, often using terms representing fractional regeneration efficiency. This modeling is crucial for designing effective catalyst management strategies in industrial operations.
Catalyst Life Cycle Examples
Catalyst life cycles are critical in various industrial processes. Examples highlight practical applications and how catalysts operate across different phases, ensuring efficiency and sustainability in chemical reactions.
Real-World Catalyst Life Cycle Examples
Understanding catalyst life cycles through real-world examples provides valuable insights:
- Automobile Catalytic Converters: These use catalysts to convert toxic gases into safer emissions. Initially activated during production, they facilitate reactions such as the conversion of carbon monoxide into carbon dioxide. Over time, they may deactivate due to lead poisoning or thermal degradation, necessitating replacement or regeneration.
- Ammonia Synthesis: In the Haber-Bosch process, iron-based catalysts are crucial. They undergo activation through reduction in hydrogen, participate in the ammonia synthesis reaction, and eventually suffer deactivation from nitrogen poisoning, requiring periodic regeneration or catalyst replacement.
An oil refinery uses zeolite catalysts in fluid catalytic cracking to break down large hydrocarbon molecules into gasoline. Initially activated by calcination, they efficiently catalyze reactions for extended periods. However, coke deposition leads to deactivation, requiring periodic regeneration via steam or air treatment.
Exploring deactivation in more depth, consider the case of coke deposition on catalysts. Coke is a carbonaceous residue that blocks active sites, reducing catalyst effectiveness. The buildup rate of coke may depend on factors such as feedstock quality and operating conditions.In response, regenerating catalysts often involves controlled oxidation of coke deposits at high temperatures to restore activity. Balancing reaction rates and selectivity with deactivation kinetics is essential in optimizing the process. Advanced modeling techniques may predict deactivation patterns and assist in designing more robust regeneration cycles, ensuring catalysts remain active for as long as possible.
Deactivation Factor | Regeneration Method |
Coke Deposition | Thermal Oxidation |
Sintering | Substrate Cooling |
Poisoning | Chemical Treatment |
Monitoring operating conditions closely, such as temperature and pressure, can prolong catalyst life by preventing rapid deactivation.
Catalyst Regeneration Process
Catalyst regeneration is a crucial process in maintaining the efficiency of catalysts over their operational life span. Understanding the nuances of this process can significantly enhance the longevity and performance of catalysts.
Catalyst Life Cycle Techniques
Within the catalyst life cycle, various techniques are employed to manage the catalyst's transition between different phases. These techniques ensure optimal performance and maximum lifespan.
- Activation: Involves preparing the catalyst by processes such as reduction or calcination to make it reactive.
- Monitoring Utilization: Keeping track of the catalyst's performance during reactions to optimize operating conditions.
- Deactivation Management: Addressing catalyst deactivation through scheduled monitoring and timely interventions.
- Regeneration: Implementing techniques such as thermal treatments or chemicals to restore catalyst activity.
Regeneration processes are often modeled mathematically to optimize various parameters. Consider the example of coke deposition regeneration in catalytic cracking, where the oxidation reaction can be represented as:\[ C + O_2 \rightarrow CO_2 \]This reaction helps to remove carbon deposits, thus regenerating the catalyst surface. The kinetics of such reactions are crucial for ensuring effective regeneration and involve the consideration of temperature, oxygen flow rates, and time.
Catalyst Deactivation Phases
Catalyst deactivation is inevitable, occurring in various phases and influenced by numerous factors. Understanding these phases is key to developing strategies to mitigate deactivation and extend catalyst life.
- Physical Deactivation: Results from changes in catalyst structure, such as sintering or pore blockage.
- Chemical Deactivation: Stems from strong interactions between the catalyst and feed or by-products, leading to \textit{poisoning}.
- Mechanical Deactivation: Caused by the breakdown of catalyst particles due to operational stresses.
A proactive maintenance schedule can help catch early signs of catalyst deactivation, aiding in timely regeneration before significant efficiency loss occurs.
Consider a hydroprocessing catalyst in oil refineries. Over time, sulfur compounds present in crude oil may poison the catalyst. Regular analysis and regeneration using hydrogen treatment can help restore catalyst activity, maintaining the efficiency of the refining process and extending the catalyst's functional life.
catalyst life cycle - Key takeaways
- Catalyst Life Cycle Definition: Describes the phases a catalyst undergoes: activation, utilization, deactivation, and regeneration.
- Catalyst Life Cycle Stages: Activation, utilization, deactivation, and regeneration; essential for understanding catalyst performance.
- Catalyst Life Cycle Examples: Automobile catalytic converters, ammonia synthesis, and fluid catalytic cracking illustrate different phases in practice.
- Catalyst Life Cycle Techniques: Include activation preparation, monitoring utilization, managing deactivation, and regeneration processes.
- Catalyst Regeneration Process: Involves restoring catalyst activity using methods like thermal treatments or chemical treatments.
- Catalyst Deactivation Phases: Physical, chemical, and mechanical deactivation require different strategies to counteract efficiency loss.
Learn faster with the 12 flashcards about catalyst life cycle
Sign up for free to gain access to all our flashcards.
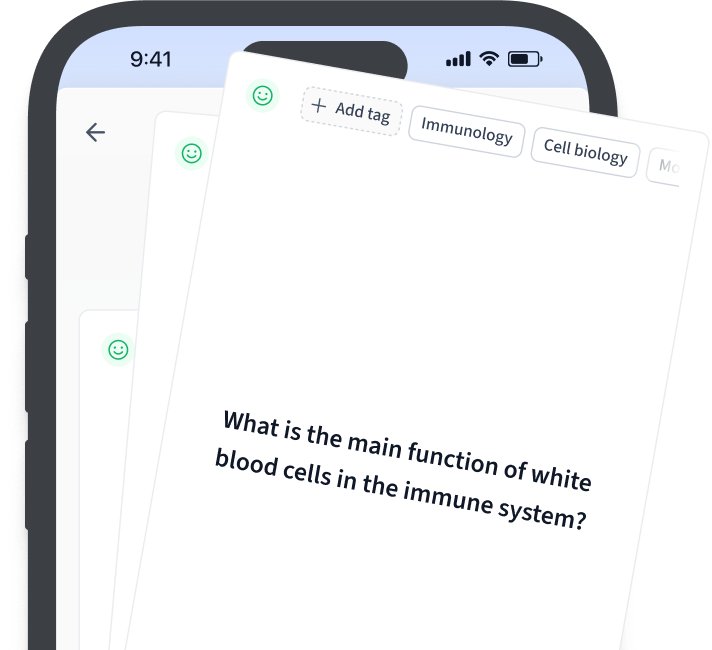
Frequently Asked Questions about catalyst life cycle
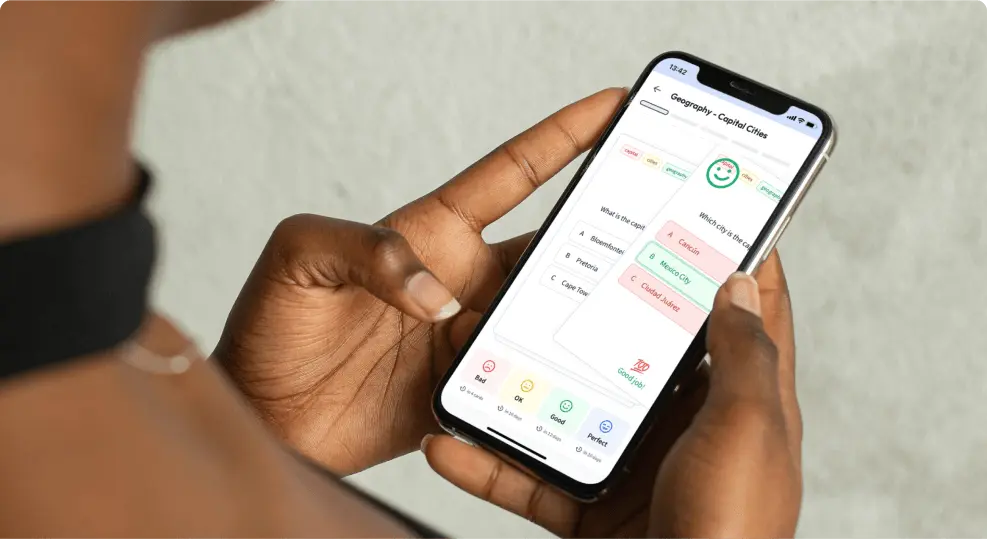
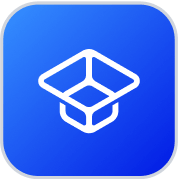
About StudySmarter
StudySmarter is a globally recognized educational technology company, offering a holistic learning platform designed for students of all ages and educational levels. Our platform provides learning support for a wide range of subjects, including STEM, Social Sciences, and Languages and also helps students to successfully master various tests and exams worldwide, such as GCSE, A Level, SAT, ACT, Abitur, and more. We offer an extensive library of learning materials, including interactive flashcards, comprehensive textbook solutions, and detailed explanations. The cutting-edge technology and tools we provide help students create their own learning materials. StudySmarter’s content is not only expert-verified but also regularly updated to ensure accuracy and relevance.
Learn more